|
|
Who |
|
|
Instrumentation (unless
completed in phase A.1) (One beam at a time)
|
|
|
|
Commission BPM intensity measurement
mode
|
|
|
|
Establish closed orbit (One beam at a time)
|
|
|
|
Integer tune measurement and correction from
difference of orbits
|
|
|
|
|
|
|
|
Closed orbit measurement and correction to
get few turns
|
|
|
|
Measurements with few turns (One beam at a
time)
|
|
|
|
Fractional tune measurement from injection
oscillation
|
|
|
|
Adjustment of chromaticity to reduce
de-coherence
|
|
|
|
Adjustment of the coupling
|
|
|
|
Fast physical aperture scans, free oscillations with BPM
intensity measurement
|
|
|
|
Systematic linear optics checks
|
|
|
|
Offsets between different sectors
(Interleaved)
|
|
|
.01 |
Commission interleaved injection |
OP/CO |
1 |
|
Correction of the orbit to 1 mm r.m.s.
|
|
|
|
Correction of the Bdl sector-by-sector down to few 10-4
|
|
|
|
Correction MQ-MQ offsets sector-by-sector from phase advance
measurement
|
|
|
|
RF observation equipment (One beam at a
time)
|
|
|
|
Set-up observation equipment and bunch
reference numbers
|
|
|
|
SPS-LHC Energy matching (Mostly interleaved)
|
|
|
|
Centre first turn in both rings
|
|
|
|
Measure revolution frequency for both beams
|
|
|
|
Correct (BdlSPS,
BdlLHC1,BdlLHC2,
fRF_SPSLHC)
|
|
|
|
|
|
|
|
Measure residual energy error and correct
|
|
|
|
Synchro Loop commissioning
and beam capture (Interleaved)
|
|
|
|
|
|
|
|
Adjust
syncho loop dynamics
|
|
|
|
Check
phasing of cavity sum
|
|
|
|
|
|
|
Details of activities:
Step A.2.1:
Instrumentation [One beam at a time]
-
If not already done: move screens OUT -
Commission BPM intensity
measurement mode:
Step A.2.2:
Establish closed orbit [One beam at a time]
-
Integer
tune measurement
-
Close the trajectory
-
Closed orbit
measurement and correction to get a few tens of turns
-
Repeat for
the other beam
Step A.2.3:
Measurements with a few turns
[One beam at a time]
-
Fractional tune measurement
-
Adjustment of the chromaticity
-
Adjustment of the coupling
-
Fast physical
aperture scans
-
Optional:
systematic linear optics
checks in case the lifetime is not
sufficient
-
Repeat for the other beam
Step A.2.4:
Offsets between
different sectors [Interleaved injection]
-
Commission
interleaved injection
-
Correction of the orbit
-
Correction of the Bdl
sector-by-sector
-
Correct
the relative octant-by-octant MB field offset down to
a few 10-4 (see
[6]).
-
Note: The B-field trim should
be propagated to the multipoles (at least to the quadrupoles) to
keep the correct MQ-MB tracking, in order to try keeping the
tune constant.
-
Optional:
Correction of the
MQ-MQ offsets sector-by-sector
Step A.2.5:
RF observation
equipment [One beam at a time] (see
Fig 1)
-
Keep RF OFF
-
Set up observation equipment
and bunch reference numbers
-
Set the observation memory to
trigger on "Beam In" timing
-
Observe the pick up signals (APW in Beam
Phase module and BPM in Beam Position module).
-
Adjust the gain/attenuation of the
RF front end of the Beam Position and Beam Phase modules
-
Align the signals from the 2 inputs
-
Easy for APW and Δ,Σ from BPM
-
More difficult for cavity sum as
the beam induced voltage will be very small with pilot. Coarse
adjustment must be done without beam.
-
Adjust the Frev marker
(offset in memory addressing) so that marker points to bucket 1
Step A.2.6:
SPS-LHC energy matching and Phase Loop commissioning [Mostly
interleaved
injection]
[3x8 hours]
Notes:
-
Before the energy matching
can start the beam must survive for at least 1/4 of a
synchrotron period (that is about 45 turns at nominal voltage,
that is 8MV, or about 35 turns at 16MV).
-
It is assumed that one uses a
common frequency for both beams in SPS and LHC (fSPS-LHC)
-
As a starting point for energy
matching, the beam should be centred in the SPS at extraction and in
the LHC for both rings (with RF OFF)
-
Centre the first turn in both
rings
-
RF Preparation:
-
Keep the Synchro Loop ON
-
Keep Phase Loop OFF
-
Keep Radial Loop OFF
-
Keep RF OFF
-
Use pilot beam with small momentum spread
-
Centre the beam in the SPS at extraction
-
Measure the revolution
frequency frev
for both beams
-
Correct BdlSPS,
BdlLHC1, BdlLHC2, fRF SPSLHC
-
Trim fRF SPSLHC, the
LHC integrated fields for beam 1 (BdlLHC1) and beam 2
(BdlLHC2), via the COD correctors only
(see
[1] and
[3]),
and the magnetic field at extraction in the SPS (BdlSPS).
-
Notes:
-
There will be a radial offset at
extraction in the SPS if CLHC differs from 27/7 CSPS.
-
There will be a radial offset in the LHC
after capture only if the two rings have a different
circumference (~0.1 mm for ~1.5 mm difference).
-
1 cm length difference
corresponds to about 1.5 mm radial
offset, that is to about 150 Hz
-
Commission the RF
Phase Loop (see Fig 1)
-
RF preparation:
-
Switch the RF ON (with 1MV/cavity
[14]).
-
Switch the Phase Loop ON
-
Switch the Synchro Loop OFF
-
Observe the phase between the bunch and the
total voltage Vt on the
first few turns (by using the Phase Loop phase discri ΔФphase).
-
The phase loop will lock, but as the stable
phase is wrong the beam will be accelerated or decelerated.
-
Because the RF is ON and the phase is wrong,
in the worst case (90 degrees error) the beam will be
driven out of the chamber in 140 turns or 14 ms
-
Adjust the RF phase loop gain
to get a decent response
-
Look at the Synchro Loop phase discri output ΔФsync.
-
As we are on the Phase Loop, the beam
imposes the RF frequency.
-
The beating of the Synchro Loop phase discri
is thus the injection frequency error.
Fig. 2: SPS phase loop and synchro
loop discri at injection. Synchro loop off. Wrong frequency. [12].
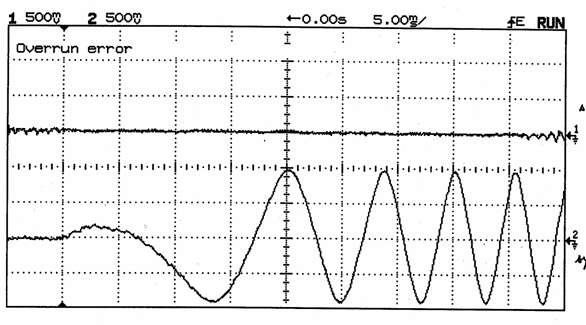
Fig. 3: SPS phase loop and synchro
loop discri at injection. Synchro loop off. Correct frequency but wrong
phase. [12].
-
Coarse adjustment of the Stable Phase to get a
constant beat frequency. The beam is neither accelerated nor
decelerated.
-
Then adjust the injection phase to cancel the
transient in the Phase Loop phase discri output ΔФphase.
-
Measure the beat frequency, that is the frequency
error at injection
-
Measure
residual energy error and correct
-
Measure the residual momentum
mismatch by observing the phase pick-up signal (sinusoidal
oscillation with amplitude proportional to the momentum
mismatch)
-
Re-adjust BdlSPS,
BdlLHC1, BdlLHC2, fRF SPSLHC
Step A.2.7:
Beam capture and Synchro Loop Commissioning [Interleaved
injection]
(see Fig 1)
[2x8 hours]
[One beam at a time]
Note:
-
Commission
Synchro Loop
-
RF Preparation:
-
Keep RF ON
-
Leave the Synchro Loop ON at
injection
-
Keep Phase Loop ON
-
Keep Radial Loop OFF
-
Fine tune the injection phase
and the stable phase:
-
A small frequency error at
injection can be deduced from the slope of the Synchro Loop
phase discri ΔФsync.
-
Adjust Synchro
Loop dynamics [8 hours]
-
With both loops ON (Phase Loop
and Synchro Loop), measure the synchro loop step response
-
Adjust the synchro loop gain and
phase advance to fine tune the response
-
Optional
at this stage: As this depends on the RF voltage via
synchrotron frequency, it should be done at different voltages.
This fine tuning at different RF voltages should be done before
Phase A.8 (snapback and ramp).
-
Optional
at this stage:
Check phasing
of cavity sum [8 hours]
Note: should be done before
before Phase A.8 (snapback and ramp) AND before increasing
intensity.
-
Now try to capture the beam with one cavity at a
time
-
Observe synchro loop phase discri output ΔФsync
after transient
-
If non-zero, fine-tune the delay in Cavity Sum
for that cavity
-
Repeat for second ring
-
Fine tune capture [2x4 hours]
-
Voltage matching SPS-LHC:
-
Observe 400 MHz component of bunch intensity
using Bunch Phase module (or APW peak).
-
Minimize quadrupole oscillations
-
Measure the bunch profile at injection (using a
scope in SR4 or OASIS)
-
Optional at this stage: Measure the lifetime (using a scope in SR4 or OASIS).
Should be done before Phase A.8 (snapback and ramp).
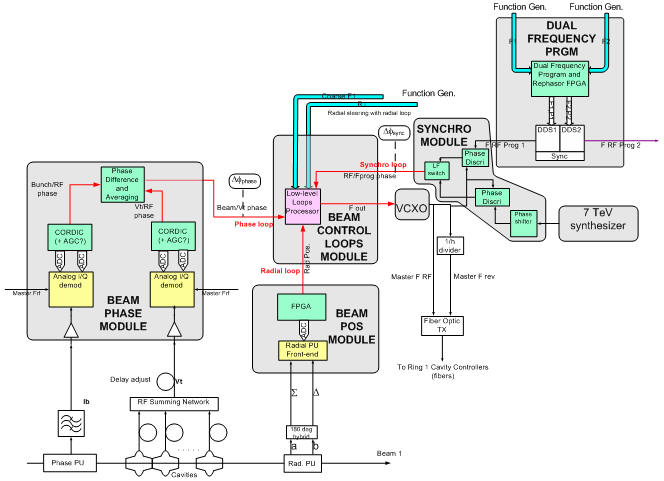
Fig. 1: Low level RF Beam Control
Loops [12].
Notes on figure 1:
-
Injection frequency, injection phase and
stable phase will be adjusted by observing two signals: ΔФphase
and ΔФsync
.
-
A function sets the RF frequency on the
injection plateau and through the ramp, via the Dual Frequency
Program
-
The 7 TeV synthesizer replaces the
frequency program during physics
-
The VXCO generates the RF sent to the
Cavity Controllers
|